Four hundred years since Galileo’s astronomical discoveries
By Hector Cordon
15 August 2009
The International Astronomy Union and the United Nations Educational, Scientific and Cultural Organization (UNESCO) have designated this year as the International Year of Astronomy 2009 in tribute to the 400th anniversary of the astronomical discoveries of Galileo and Johannes Kepler, whose A New Astronomy was published in the same year as Galileo’s discoveries with the telescope.

Galileo is primarily recognized for his use of the telescope to demolish the centuries-old Aristotelian-Ptolemaic (earth-centered) view of the universe and the resultant clash with the Catholic Church. His 1609 accomplishments include significant improvements to the telescope, detailed sketches of the Moon’s features, followed by, in 1610, the discovery of the four moons of Jupiter (now known as the Galilean moons) and the odd shape of Saturn (the telescope was still too primitive to discern the rings), his observations of sunspots, and the discovery that Venus went through phases similar to those of the Moon
Both Galileo and Kepler made tremendously original and profound contributions toward understanding the physical laws of the universe—Kepler, most famously with his three laws of planetary motion. A New Astronomy contains a detailed calculation of Mars’s orbit and Kepler’s first two laws of planetary motion.
His first law established that planetary orbits are ellipses with the Sun at one focus of the ellipse, and, the second law, obeying a simple mathematical rule, showed that a line joining any planet in its orbit to the Sun would sweep out equal areas in equal times. Kepler’s findings were only possible due to Danish astronomer Tycho Brahe’s extraordinarily precise (for its time) measurements of planetary orbits, which, in turn, were possible due to the greater precision of the instruments available to him. Kepler’s third law, added in 1619, provides a precise mathematical depiction of the relationship of the distance from the sun of a planet to its orbital period. Newton, in his Principia Mathematica of 1687, incorporated this finding into his law of universal gravitation.
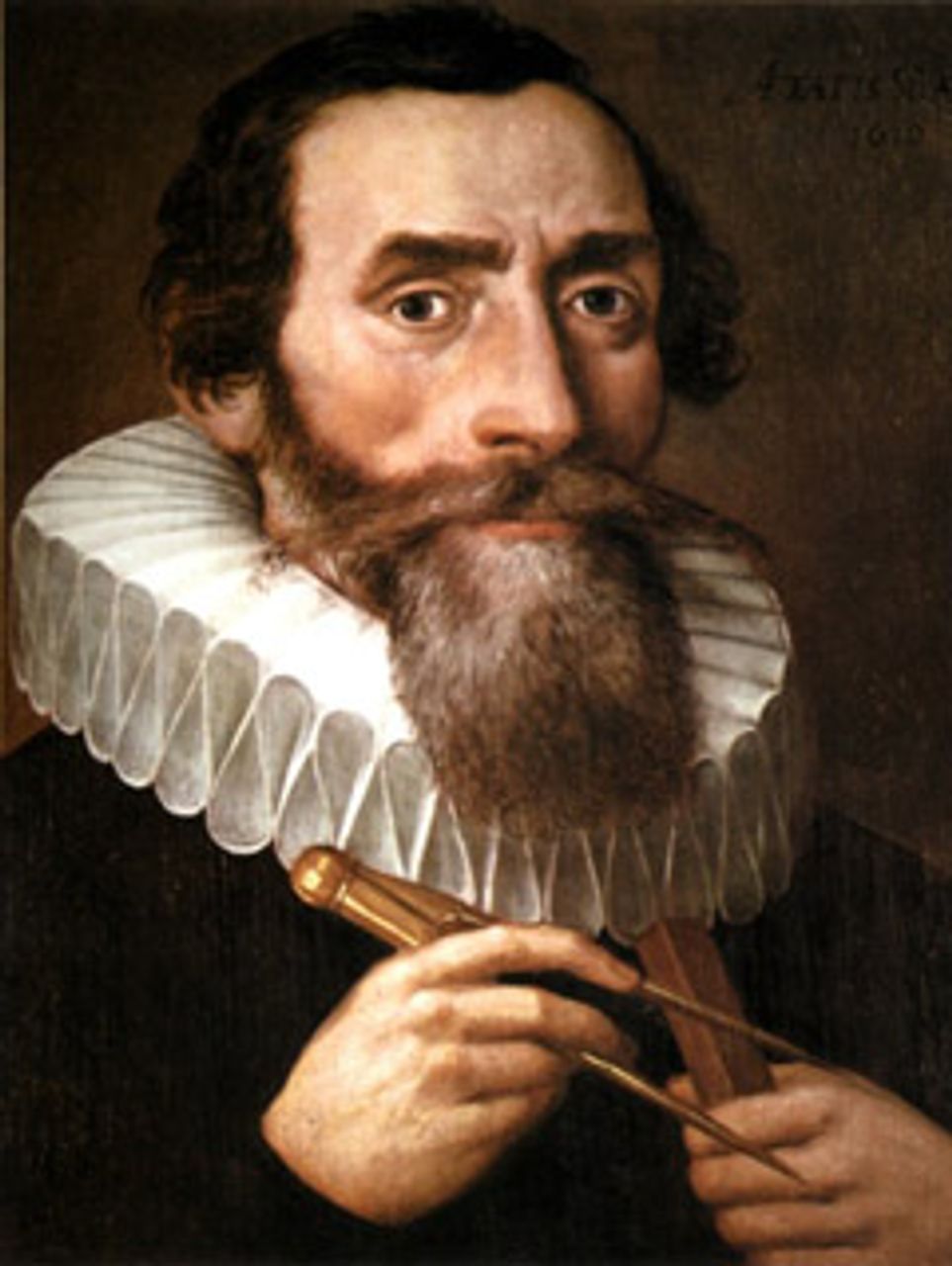
Both men were responsible for revolutionizing not only astronomy, but also many areas of science. Galileo created a science of motion and the strength of materials, while constructing new instruments and making improvements to others. Kepler made gigantic strides in the theory of optics and problems in geometry. It was Kepler’s theoretical work in optics that allowed him to explain the principle of the telescope, leading to improvements in its design. His effort placed Copernicanism on a firm mathematical foundation. Their body of work laid the basis for many scientific fields today.
The 400th anniversary of Galileo’s insight of using what was originally called a spyglass and subsequently renamed the telescope to observe and understand the heavens has occasioned some comment in the media; most of it is of a celebratory and superficial nature, with little, if any, that provides an understanding of what Galileo’s accomplishments represent historically and scientifically.
What united Galileo and Kepler, in addition to their intellectual independence and scientific accomplishments, is that both became, early on, adherents of Copernicanism—the outlook that the sun, not the earth, occupied the center of the universe—Kepler, while still a student and Galileo around 1595 as a professor in Pisa. At Tubingen, where Kepler began his studies, one of his teachers was Michael Maestlin, a talented astronomer and secret follower of Copernicus. Through Maestlin, Kepler read Copernicus’s 1543 book, Six Books Concerning the Revolutions of the Heavenly Orbs and became a convert. In a letter to Kepler in 1597, Galileo professed his long-time support for the heliocentric model “because it reveals to me the causes of many natural phenomena that are entirely incomprehensible in the light of the generally accepted hypothesis.”
Maestlin’s secrecy was essential due to the persecution by the Catholic Church of those who supported Copernicus’s heliocentric cosmology. Heliocentrism contradicted a central tenet of Church theology, the scripturally mandated, earth-centered universe. In the thirteenth century, Thomas Aquinas had interwoven Ptolemy’s geocentric model into Catholic theology along with Aristotle’s immutable (never-changing) heavens—in other words, an ordering to the universe that paralleled the ordering of a rigid feudalistic society. Any challenge to geocentrism was therefore also a challenge to the authority of the Church.
Despite the lack of empirical proof, both Galileo and Kepler sensed intuitively that Copernicus’s model provided a more substantive explanation for the workings of the universe than the Ptolemaic system. The arrival of Tycho’s supernova in 1572 and Kepler’s supernova in 1604, along with the appearance of various comets, showed that the heavens were far from immutable, further contributing to the erosion of Church authority.
Notwithstanding their rejection of the Church-defended geocentrism, both remained staunch adherents of religion. Kepler sought to illustrate God’s presence through the explicit workings of the universe. Galileo took a more distanced approach, seeking to reveal nature’s laws, and therefore God’s works, through the use of mathematics. That neither was able to break with superstition is due to the dominant atmosphere of religious obscurantism of the time; even so, their historic contribution is that they developed a rational method that directly challenged the Church.
That both Galileo and Kepler were deeply religious—not an unusual characteristic in a time when all schools were run by the Church—does not constitute an argument in favor of the Church-supported reconciliation of science and religion nor the outlook, championed by the late paleontologist Stephen J. Gould, of “non-overlapping magisteria.” Both men were also practicing astrologers, which does not give any boost to the credibility of that pseudoscience. In fact, science and religion are two wholly opposed philosophical outlooks.
The Church, for its part, instinctively recognized the implications of this new world outlook and responded with threats of imprisonment, torture and death—a response unerringly guided by centuries of experience in defense of power, wealth and order. Making good on their threats, the Church waged a virtual campaign of terror to suppress the inherent tendency of this new science to escape its control. “Over a ten-year period, Pope Clement VIII banned Patrizi’s Nova philosophia, Telesio’s De rerum natura, and the complete works of Giordano Bruno and Tommaso Campanella, initiated proceedings against Giambattista Della Porta and Cesare Cremonini, and condemned Francesco Pucci to death, imprisoned Campanella, and had Bruno burned at the stake.” (1)
Kepler was eventually sentenced to what can be considered relatively mild punishment: excommunication from the Lutheran Church. Galileo’s dramatic encounter, however, became transformed into a major battle in the continuing conflict between science and religious superstition.
Galileo Galilei
Albert Einstein called Galileo “the father of modern physics—indeed of modern science altogether.” And Stephen Hawking has stated, “Galileo, perhaps more than any other single person, was responsible for the birth of modern science.”
Galileo’s chief contribution to science is his championing of the experimental method to quantify motion, in opposition to the dominant Aristotelian philosophy. Galileo proposed as early as 1589, in his unpublished manuscript On Motion, the need for the application of experience to determine the truth of a proposition. While he was not the first to make this proposal—Copernicus expressed a similar view—Galileo, in contrast, sought to support his theoretical work with real-world experiments.
Galileo was born in Pisa, Italy, on February 15, 1564. His family moved to the urban environment of Florence in 1574, where he later joined them. Florence (where one of the earliest workers’ uprisings in history occurred when wool workers revolted in 1378, in the Ciompi Revolt) was a remarkably large city for its time, with a major mercantile, trade and manufacturing economy. The city was economically and politically dominated by the powerful Medici family, whose members played significant roles as patrons of the arts and sciences, including support for Galileo’s work.
This was the period of time—between the Renaissance and the Enlightenment—when the Catholic Church had launched its Counter-Reformation in reaction to the Protestant Reformation. The resentment of the newly developing centralized political states and the emerging middle class toward the Vatican’s monopoly of power and wealth found its ideological reflection within the Church, giving rise to a reform movement, or more precisely, multiple movements organized primarily along national boundaries: Luther in Germany, Calvin in France, Zwingli in Switzerland and the split that formed the Anglican Church in England. The Reformation expropriated local Church property, exacting huge losses of wealth, land, and schools, as well as influence. The Catholic Encyclopedia cites—with no apparent irony—the secular support for this movement. “The history of the Reformation shows incontestably that the civil power was the chief factor in spreading it in all lands, and that in the last analysis it was not religious, but dynastic, political and social interests which proved decisive.” (2)
Concurrently, the development of the printing press—invented just 33 years before the birth of Copernicus—allowed for the inexpensive and rapid circulation of new ideas and new literature (Shakespeare was born the same year as Galileo, while the first modern novel, Cervantes’s Don Quixote, was published in 1605), giving rise to further challenges to the authority of the Church. From within and without, the Catholic Church faced the consequences of the eruption of a new social force, the bourgeoisie. Frederick Engels, Marx’s co-thinker, said of the era, “It was the epoch which brought into being the great monarchies in Europe, broke the spiritual dictatorship of the Pope, evoked the revival of Greek antiquity and with it the highest artistic development of the new age, broke through the boundaries of the old world, and for the first time really discovered the world.” (3)
This, then, is what determined the Church’s response to Galileo.
In 1581, Galileo began his advanced studies at the University of Pisa, originally for a medical degree due to his father’s desire, but then switching to his preferred field, mathematics. According to his first biographer, Vincenzo Viviani, while a student in Pisa, Galileo first formulated the isochronism (uniform in time motion) of the pendulum. To Aristotelian physics, with its theory that objects inherently sought the center of the earth, this phenomenon was inscrutable. Galileo, however, worked on problems from an Archimedean, or quantitative, approach. His propensity to interpret real-world phenomenon in mathematical terms inevitably led to a rejection of the Aristotelian method.
Beginning in 1589, Galileo was appointed professor of mathematics at the University of Pisa. Three years later, the university refused to renew his contract due to the irritation he caused the earnest Aristotelian professors who bore the brunt of Galileo’s scorn for those who “think that our intellect should be enslaved to that of some other man.” (4) He then obtained an appointment to the University of Padua in the Republic of Venice in 1592 as chair of mathematics, where he taught geometry, mechanics, and astronomy for the next 18 years.
While in Pisa, Galileo had worked on problems of motion, a field in which he felt he made his greatest contributions. In defining what forces make movement possible, Galileo wrote in On Motion, “...it seems that Aristotle also adduces this cause [supreme providence] in Physics, book VIII, text 32, [255b15-17], when, asking why heavy things and light ones are moved towards their proper places, he supposes that the cause is because they are by nature suited to be carried somewhere, that is, the light upward, and the heavy downward.” Dissatisfied with this explanation, Galileo applied his new method, experience; he experimented with bronze balls rolled down an inclined plane. Contrary to Aristotle, the balls, regardless of differing weights, descended with the same acceleration.
Additionally, Galileo determined what that rate was: the distance traveled is directly proportional to the square of the elapsed time. “Whatever distance the objects covered in one instant—measured as a pulse beat, a sung note, the weight of water that dripped from Galileo’s timing device—by the end of two such instants it would travel four times as far. After three instants, it wound up at nine times the initial distance of descent; after four instants, 16 distance units—and so on, always accelerating, always covering a distance determined by the square of the time passed.” (5)
Modern science had indeed arrived; mathematics was now applied to motion. However, it arrived without a reliable unit of measure or an accurate clock. Hence, Galileo was forced to devise his own standards, using his heartbeat or dripped water to time events, and millet seeds, hand span or arm length to measure distances.
Galileo also considered what happened once the ball left the inclined plane. At this point, the effect of gravity is constant—ignoring friction, the ball would continue to move in a straight line and in a constant uniform motion, in other words, demonstrating inertia: “A body moving on a level surface will continue in the same direction at constant speed unless disturbed.” This insight was one of Galileo’s more important findings, and combined with his law of free-fall led to his description of a projectile as always describing a parabola. It is a version of inertia that Newton used to develop his First Law of Motion.
By 1609, news arrived of a recent invention by, some say, Dutch optician Hans Lipperhey, who had fixed two lenses into a tube and with this was able to magnify the images of distant objects. Galileo grasped the principles involved and proceeded to build his own telescope. Initially using purchased lenses, but subsequently grinding his own, he rapidly increased viewing power from 3 times to 30 times magnification. Galileo was thus able to observe Earth’s moon with unprecedented detail
He found that it was not Aristotle’s perfect celestial orb but had “protuberances and cavities,” much like the Earth. The planets had disks and were not star-like points of light; the Milky Way was composed of an endless number of stars; and “four planets swiftly revolving about Jupiter at differing distances and periods.” (6) The discovery of the phases of Venus, Jupiter’s moons and sunspots provided support for heliocentrism as well as a sense of vindication for Galileo.
Realizing the implications of his observations for the Copernican model, Galileo gathered his notes, sketches of the Moon and of Jupiter with its moons, and his charts of the many new stars now visible in the Pleiades and Orion star cluster, and wrote his celebrated Starry Messenger. Its publication in 1610 generated considerable public interest—analogous to the Apollo landing on the Moon 360 years later—circulating as far away as China. Much of the popularity was due to Galileo’s writing it in the Italian language, as opposed to the Latin favored by the academics, and thus making it widely accessible.
The response of the Church was cautious, but inevitable, culminating in Galileo’s first encounter with the Congregation of the Holy Office in 1616. The same inquisitor, Robert Cardinal Bellarmino, the so-called “hammer of the heretics,” who concurred in sentencing Giordano Bruno to be burned at the stake in 1600, now condemned the Copernican viewpoint as “absurd in philosophy, and formally heretical.” Bellarmino, who had followed Galileo’s work since 1611, issued the notorious Edict of 1616, directed by Pope Paul V, forbidding Galileo from defending Copernicanism “in any way whatever, either orally or in writing” and ordering him to abandon it “completely.”
Now in his fifties and in ill health, Galileo abandoned his public support for Copernicanism, but continued with his astronomical observations. He worked unsuccessfully to find a method whereby Jupiter’s moons could be used to determine longitude at sea, and he tracked three comets in 1618. He wrote The Assayer in 1623 as a polemic against Jesuit mathematician Orazio Grassi, arguing, incorrectly, that comets were a play of light and not real objects.
The book, however, is considered his scientific manifesto as it contains wider arguments on the nature of science itself, brilliantly expressed in Galileo’s famed statement, “Philosophy is written in this grand book—I mean the universe—which stands continually open to our gaze, but it cannot be understood unless one first learns to comprehend the language and interpret the characters in which it is written. It is written in the language of mathematics, and its characters are triangles, circles, and other geometrical figures, without which it is humanly impossible to understand a single word of it; without these, one is wandering around in a dark labyrinth.” (7)
Galileo outlines here a succinct exposition of the materialist approach indispensable to understanding nature. What he championed, quite consciously in opposition to Aristotle’s approach, was a method of thought that sought to accurately reflect the laws of matter in man’s thinking.
With the 1623 election of his friend and supporter, Maffeo Barberini, to head the Church as Pope Urban VIII—to whom his book The Assayer was dedicated—Galileo felt that he might be able to lift the prohibition placed on him by the Edict of 1616. Though the new pope refused the request, he did encourage Galileo’s newest project, a book contrasting the Ptolemaic and Copernican system, but warned him to be careful to treat heliocentrism only as a mathematical hypothesis. The publication of Dialogue on the Two Chief World Systems in 1632, despite the warning, became the catalyst for a second confrontation with the Church hierarchy.
Accused of defending heliocentrism in violation of the Edict, Galileo was summoned to appear before the Inquisition to face charges of heresy. Shown the instruments of torture and invited to recant, Galileo confessed to going beyond the restrictions of the Edict. The Inquisition found Galileo “vehemently suspect of heresy” for advocating Copernicanism and required him to publicly “abjure, curse and detest” this belief. His Dialogue was banned and placed on the Index of Forbidden Books. The Inquisition banned all books previously written by Galileo and, unknown to him, all future books he might write. His initial imprisonment was changed to house arrest, where Galileo was to spend the rest of his life, dying in 1642.
While under house arrest Galileo wrote his most important and final book, the 1638 Discourses and Mathematical Demonstrations Relating to Two New Sciences, summarizing his 30 years of work on motion and on the strength of materials. “The task he set himself in the Two New Sciences was to develop concepts, methods of calculation and measurement...to arrive at a description of the motion of objects in rigorous mathematical form” (8)
In a letter to his long-time friend Elia Diodati, Galileo described Two New Sciences as “superior to everything else of mine hitherto published.” Publication of the book was hindered as a result of his condemnation for heresy by the Holy Office, but the manuscript was finally smuggled out of Italy to the Netherlands where it was printed.
The person of Galileo represented the rare combination of acute observational ability, technical genius, mathematical brilliance and avid intellectual curiosity. His rise to the pinnacle of science was the result of an intersection of these traits with the impetus of a new social order seeking to exploit a mostly unknown world. Engels described this period in these words: “The greatest progressive revolution that mankind had so far experienced, a time which called for giants and produced giants—giants in power of thought, passion and character, in universality and learning. The men who founded the modern rule of the bourgeoisie had anything but bourgeois limitations. On the contrary, the adventurous character of the time inspired them to a greater or lesser degree. There was hardly any man of importance then living who had not traveled extensively, who did not speak four or five languages, who did not shine in a number of fields.” (9)
1. Paolo Rossi, The Birth of Modern Science, Blackwell Publishers, 2001, p. 80
3. Frederick Engels, Dialectics of Nature, p. 184
4. Galileo, The Assayer, Drake, 1957, p. 238
5. Dava Sobel, “Galileo, His Place in Science,” Public Broadcasting Service
6. Galileo, Starry Messenger, Introduction
7. Galileo, The Assayer, Drake, 1957, p. 237
8. Gerald James Holton, Stephen G. Brush, Physics, the Human Adventure, Rutgers University Press, p. 82
9. Frederick Engels, Dialectics of Nature, p. 2
No comments:
Post a Comment